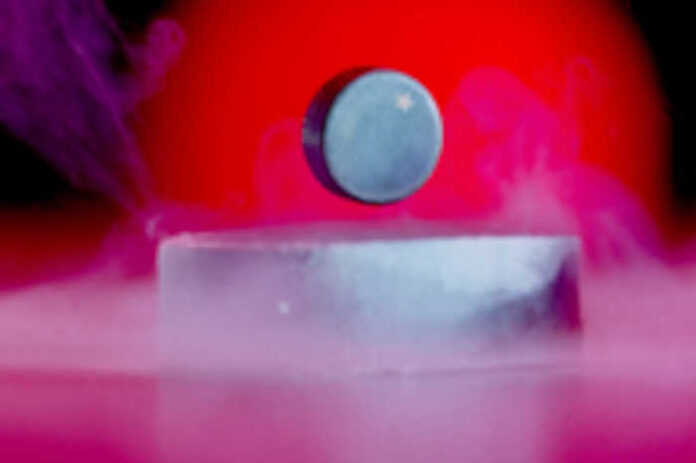
2011 marks the 100th anniversary of the discovery of superconductivity, the ability of some materials to conduct electricity with zero energy loss when cooled to extremely low temperatures.
This fundamental property of some materials was discovered by Dutch scientist Heike Kamerlingh Onnes at Leiden University.
Around 4 p.m. on April 8, 1911, he cooled mercury to 4.19 degrees Kelvin and found his instruments suddenly measured zero resistivity – no electricity was lost as heat or friction. He received the Nobel Prize in Physics for this accomplishment two years later. This phenomenon is called superconductivity. An electric current in a loop of a superconductor could, theoretically, last forever; in experiments they have been observed to last for years.
For decades after Onnes’ discovery, no one understood why superconductors worked. Einstein puzzled over it. The great quantum physicist Richard Feynman was stumped.
Superconductors have already given us MRIs, particle accelerators and better cell phone reception, but further improvements could revolutionize technology as we know it. The trouble is, they still hold some of the greatest mysteries in physics.
“All of the great minds had a go at figuring out the theory behind superconductors,” said Argonne Distinguished Fellow and materials scientist Mike Norman. “But no one figured it out until nearly 50 years later, in 1957.”
That year, a trio of physicists from the University of Illinois proposed a neat theory called BCS theory (for the three: Bardeen, Cooper and Streiffer). A current of electricity consists of electrons moving along a material. In a normal material, they must navigate the lattice-like structure of the material—and every time an electron bounces off one of the pillars of the lattice, it’s lost as heat. But in a superconductor, the electrons are bound together in “Cooper pairs”, which sail through the lattice.
The problem, of course, is that mercury only works as a superconductor at the frigid temperature of nearly -500°F. In the following years, a few other materials were discovered as superconductors, but none could operate above -420°F or so.
“Then, 25 years ago, the physics world received a great shock,” Norman said. “A team from a small Switzerland lab demonstrated what we call high-temperature superconductors.”
These superconductors could operate at “high” temperatures—that is, high compared to classical superconductors; the new conductors worked up to 100°K, or -280°F.
“That may not sound like a big difference,” Norman said, “but you can cool a conductor to that temperature using liquid air, which is much, much cheaper and easier than using liquid helium.”
The discovery, though useful, presented another problem to physicists: We don’t know why high-temperature superconductors work. Although hundreds of theories have been proposed, thousands of papers written, and physicists from every area have tried their hands at it, there is still no accepted theory of high-temperature superconductivity.
Harnessing superconductors
Superconductors are an integral component of MRI medical imaging machines and, more recently, cell phone towers. “Companies discovered that using superconductors at cell base stations allowed them to cram much more signal into a given bandwidth,” Norman explained. Superconductors magnetic properties are also used in the Large Hadron Collider in Europe, the world’s largest particle accelerator.
But superconductors’ demands have made them hard to use extensively. “If they could be laid as wire in power grids, we’d save an astounding amount of power,” Norman said—today as much as 10% of electricity is lost along power lines. But in addition to temperature demands, certain physical properties of high-temperature superconductors make them finicky.
Today’s high-temperature superconductors are arranged much like mica the shiny rock that flakes off in pieces when scratched with a fingernail. Superconductors have long layers that conduct electricity perfectly in one direction, but as soon as the wire is bent, the material starts to lose this property.
The challenge for scientists is to find a solution to that problem: a superconductor that is isotropic, or keeps its performance even when twisted into a coil.
“That discovery would revolutionize technology,” Norman said. “We’d get much improved electronics, power lines, even electric motors—our best engines are less than 50 percent efficient because so much energy is lost as heat. That’s why a motor is hot when you put your hand on it.
“If we could get the cost down, it could be the next biggest revolution in technology since the transistor.”
Argonne is co-leading a U.S. Department of Energy – ‘Energy Frontier Research Center’ on superconductivity, along with the University of Illinois and Brookhaven National Laboratory. The Center is charged with tackling big challenges in superconductivity, including the goal of isotropic superconductors.
Until then, superconductors will continue to beguile the scientific world.